The shape memory of polymers is a property that allows an object deformed by means of a physical stress to recover its initial shape under the effect of another stress. Most commonly, the initial stress is mechanical and is applied at a temperature above room temperature, while the return to the initial shape is achieved by heating. Only this type of shape memory will be discussed below.
This property is intrinsic to the macromolecular nature of polymers and can be partial or total depending on the topology of the polymer making up the object under consideration.
In the most common case, which is that of the initial deformation of the object under the combined effects of temperature and mechanical stress, the phenomenon can be interpreted by means of a set of more or less sophisticated theories.
The plasticity of polymer materials is their ability to deform irreversibly under the effect of mechanical stress. Deformation results from the movement (creeping according to Gennes’ theory) of chain segments that will progressively disentangle and align themselves in the direction of the stress, after successive breaks and re-establishments of molecular interactions. The mobility of these segments implies the existence, within the material, of spaces free of matter, called free volume. According to the corresponding theory, the fraction of free volume allowing the movement of the segments is 0.025. Below this value, the polymer chains are fixed in their conformation and the material is in the glassy, rigid state. Above this value, the free volume allows the mobility of the chain segments and the material is plastic. The temperature at which the material is deformable is the glass transition temperature (Tg). As the temperature rises, the material expands, which corresponds to an increase in the free volume and thus to an increase in the mobility of the chains.
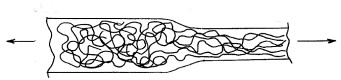
Figure showing the stretching of chains under the effect of mechanical stress.
Note 1: The glass transition temperature (Tg) does not have a well-defined value because it depends on the speed at which the mechanical stress is applied. If the speed at which the stress is applied is fast, there is no time for the chains to unravel and the sample breaks. If the stress is applied slowly, disentanglement has time to take place and the specimen deforms; it is in the plastic state.
Note 2: The phenomenon of partial shape memory, uses the non-instantaneity of disentanglement: if the applied stress is released after a very short time, the chains tend to return to their maximum entropy conformation, and the sample returns to its initial shape. Thus, depending on the duration of the stress, the shape memory is more or less good.
Total Shape Memory utilizes the ability to deform a sample above its (Tg) and, by cooling it below its (Tg) without giving the chains time to return to their maximum entropy conformation (Note 2), to freeze their constrained conformation. The treated sample only regains its initial shape after heating above the glass transition temperature which allows the chains to regain their maximum entropy conformation.
However, with monodimensional polymers that are endowed with plasticity, the memory is not perfect because, no matter how quickly the constrained conformation is blocked, some chain segments manage to increase their entropy by shrinking. Perfection can only be achieved with three-dimensional polymers. In this case, the sample consists of only one macromolecule and the relative displacement of the chains is not to be considered. An example of this situation is given by a vulcanised (three-dimensional) elastomer (Tg ~ -80°C), which is immersed in liquid nitrogen (-180°C) after deformation, and which regains its perfect initial shape on heating.
To sum up, the shape memory of polymers results from the ability of the constituent chains to regain their maximum entropy conformation.
Only materials that are to be used at or near room temperature or at human body temperature for biomedical engineering applications are considered here.
- One-dimensional glassy polymers
Polymers in the glassy state are completely amorphous and their mechanical properties are determined by the difference between the temperature of use (blocking or releasing the shape) and the glass transition temperature. The glass transition temperature can be adjusted by means of plasticizers; their proportion in the material is adjusted to the desired Tg value. Stressed forming is carried out at a temperature slightly above Tg and then cooled down abruptly to block the shapes. For this type of polymer, the quality of the memory is very dependent on the molar mass, whose values are sometimes desirable and are of the order of or above 106 g/mol.
For biomedical engineering applications, the Tg value should be slightly lower than the human body temperature, i.e. ~35°C.
- One-dimensional semi-crystalline polymers
For this type of polymers, the crystalline zones (crystallites) are generally the ones that ensure the main part of the cohesion of the system. Stress shaping and shape release therefore require the sample to be brought above the melting temperature of the crystallites. This temperature is much higher than Tg and it is very difficult to block the conformations before total cooling. This is the case of polyethylene for which it is desirable to cross-link after stress shaping (see below).
- Three-dimensional polymers
Due to their topology, all three-dimensional polymers possess shape memory at all temperatures. In order to be usable in 2 forms at a given temperature, they have the same constraints as the monodimensional ones with regard to Tg and give rise to a total recovery of the relaxed shape. However, in order to be able to deform during constrained shaping, they must have a sufficiently high molar mass between network nodes.
Cross-linking may result from the average valency of the reaction mixture at the time of polymerisation, or from cross-linking of the stressed material by physical or chemical means. For example, the reduction in diameter by heating a polyethylene pipe can be obtained by prior irradiation γ of this same pipe expanded above its melting temperature.
The applications of these shape memory polymers are important, but new ones are still to be discovered.
For more information about this product, please contact us !