Chemistry and plastics have always had a negative connotation. Associated with industrial accidents or pollution, it is however difficult today to do without them. Omnipresent in our daily lives (transport, textiles, electronic devices, construction, packaging, etc.), the chemistry of plastics, or polymers, also aims to improve the comfort of human life through the development of medicines. However, its impact on the environment has led scientists to change their way of thinking and has led them to conceive chemistry in a different way, in order to adapt it to the current challenges of preserving the environment and human health. Samuel Dufreche, R&D engineer at PolymerExpert, explains the challenges of green chemistry.
In a perpetual quest for performance, chemistry has allowed humanity to develop rapidly and to improve the comfort of life. However, these technological advances have had heavy impacts on the environment. Indeed, in 1950, the manufacture of plastics did not care about the durability of materials. In 70 years, more than 9 billion tons of plastics have been produced, and less than 10% of these have been recycled.1 Moreover, the chemical sector now occupies an important place in global carbon emissions, so it is an area where action can lead to significant improvements on a global scale.
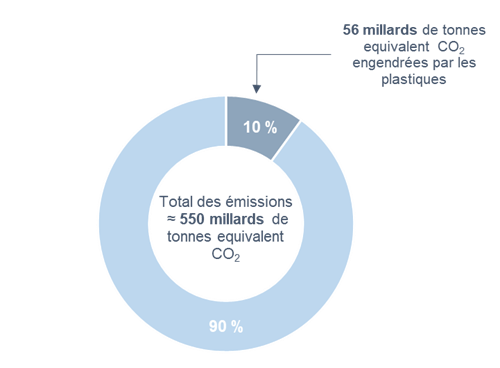
It is according to this observation that green chemistry was conceived in the 1990s. The objective for chemists Anastas and Warner was to reduce the risks generated for users during chemical practices but also to reduce the harmful impact on the environment of the products used and their waste. They established twelve principles to be followed (Figure 2).
All of these principles aim to allow the development of the chemical industry while respecting the health of operators, ensuring the quality of the environment and the health of consumers.
The best known example of “Green Chemistry” is the production of ibuprofen. For a long time synthesized in six steps with a low atomic use (the production processes generated nearly 60% of waste), the production routes have been rethought. Thus, the scientists succeeded in obtaining the same product in only 3 steps, with an atomic use of 77% (the 23% remaining correspond to a by-product which is revalorized afterwards). The implementation of this green process has reduced waste production and energy costs, without diminishing the capabilities of the initial product.
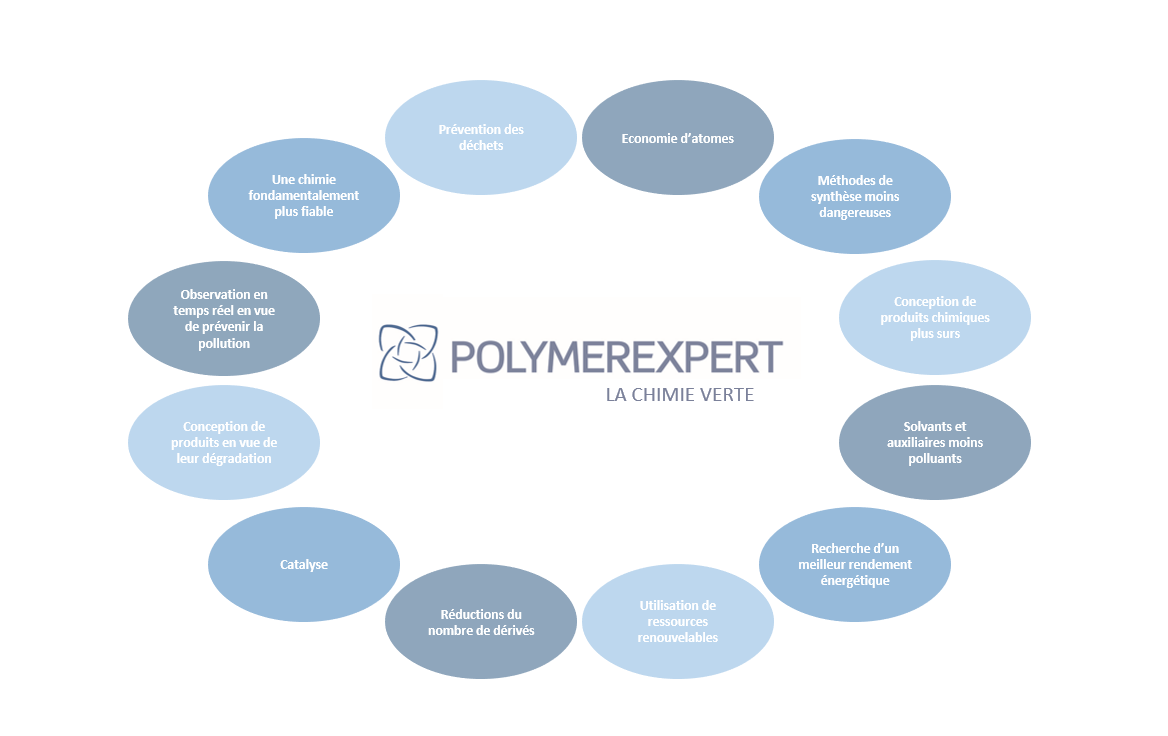
Designing according to the principle of green chemistry means reviewing and rethinking the way we work, thinking about all the possible alternatives, and finding the one that will make it possible to obtain a polymer material while limiting the amount of waste produced, energy costs, ensuring consumer safety and limiting the impact on the environment.
At the laboratory research level, large quantities of solvents are used. A reflection on a better management of the solvents to be used has led scientists to develop in 2021 a tool to facilitate this search for green solvents 2
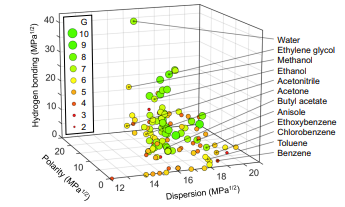
This tool takes into account two criteria specific to each solvent:
a classification of its durability calculated according to its impact on health, the dangers that it can generate by its use, its impact on the environment and the ability to treat the waste that it generates. All these criteria are converted into a score ranging from 1 to 10 (10 being the optimal score for the green solvent).
Intrinsic properties of the solvent: the solubility of a polymer in a solvent is governed by the solubility parameter according to Hildebrand, which corresponds to the square root of the cohesion energy per unit volume:
δ=√((〖∆H〗_V-RT)/V)
This parameter can be separated into three solubility parameters (Hansen): the parameter δD taking into account dispersive interactions; δP which groups polar interactions; the parameter δH which considers hydrogen interactions.
δ=√(δ_P^2+δ_D^2+δ_H^2 )
According to this Hansen’s law, each liquid can be projected into a 3D space constructed from these three parameters (Figure 3). Thus, if two solvents have a neighboring situation in this space, they will probably have the ability to solubilize the same solid. When using a solvent in the laboratory, it is therefore possible to consult alternative solvents with similar parameters and which would therefore present the same solubilizing power with less harmful effects for the user and for the environment.2
One of the major axes of green chemistry is the substitution of petrochemical products by the use of renewable resources. The challenge is to replace the properties offered by petrochemicals while remaining competitive on the market and to develop new properties that could be obtained from natural resources.
As far as polymer synthesis is concerned, cleaner polymerization methods are preferred for the development of new ingredients or materials. For example, polycondensation produces no waste with optimal atomic utilization since the only by-product is water. Polyesters have been a major research topic in the field of bio-based materials for several years. Their diversity and the great adaptability of their properties make them polymers of choice for a large number of applications. They are obtained from a wide range of monomers derived from plant resources such as cellulose, starch, vegetable oils or lignin. These polymeric materials are also designed to be biodegradable.
The challenge for the chemical industry today is to substitute all petroleum products using renewable resources without compromising performance in order to more easily encourage a change in consumer habits.3
Bibliography (1) Fuhr, L. Atlas du Plastique, faits et chiffres sur le monde des polymères synthétiques. Heinrich-Böll-Stiftung. https://fr.boell.org/fr/atlas-du-plastique (accessed 2022-11-25). (2) Larsen, C.; Lundberg, P.; Tang, S.; Ràfols-Ribé, J.; Sandström, A.; Mattias Lindh, E.; Wang, J.; Edman, L. A Tool for Identifying Green Solvents for Printed Electronics. Nat. Commun. 2021, 12 (1), 4510. https://doi.org/10.1038/s41467-021-24761-x. (3) Zhang, Q.; Song, M.; Xu, Y.; Wang, W.; Wang, Z.; Zhang, L. Bio-Based Polyesters: Recent Progress and Future Prospects. Prog. Polym. Sci. 2021, 120, 101430. https://doi.org/10.1016/j.progpolymsci.2021.101430.